Introduction
In engineering and construction, material selection is crucial to the success and safety of any project. One of the most important properties to consider when choosing materials is yield strength, particularly when working with metals like alloy steel. Alloy steel yield strength is a key factor that influences the performance, durability, and safety of structures and components. This blog will explore why alloy steel yield strength matters in engineering, its implications for various applications, and how it can be optimized for different projects.
Understanding Alloy Steel Yield Strength
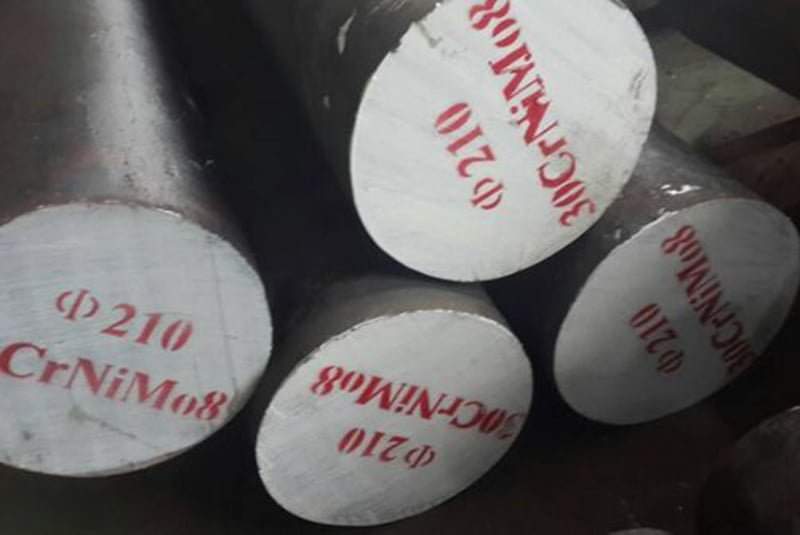
What Is Alloy Steel Yield Strength?
Alloy steel yield strength refers to the stress at which an alloy steel material begins to deform plastically. Before this point, the material will deform elastically, meaning it will return to its original shape when the applied stress is removed. Once the yield strength is exceeded, the material will undergo permanent deformation. Yield strength is measured in units of pressure, typically megapascals (MPa) or pounds per square inch (psi).
Factors Affecting Alloy Steel Yield Strength
Several factors influence the yield strength of alloy steel, including its chemical composition, manufacturing process, and heat treatment. The presence of alloying elements like chromium, nickel, and molybdenum can significantly enhance the yield strength of steel by improving its hardness and resistance to deformation. Additionally, processes such as quenching and tempering can further increase the yield strength of alloy steel.
Key Factors:
- Chemical Composition: Elements like carbon, manganese, chromium, and molybdenum contribute to higher yield strength.
- Heat Treatment: Processes like annealing, quenching, and tempering can modify the microstructure, affecting yield strength.
- Manufacturing Processes: Rolling, forging, and other processes can influence the grain structure and, consequently, the yield strength.
Importance of Alloy Steel Yield Strength in Engineering
Ensuring Structural Integrity
One of the primary reasons alloy steel yield strength is crucial in engineering is its role in ensuring structural integrity. In construction and infrastructure projects, materials must withstand various loads and stresses without deforming or failing. Alloy steel with high yield strength can bear significant stresses, making it ideal for use in bridges, buildings, and other critical structures.
Safety in Mechanical and Aerospace Engineering
In mechanical and aerospace engineering, the safety of components such as gears, shafts, and aircraft parts depends on their ability to withstand operational stresses without yielding. Alloy steel’s high yield strength ensures that these components can endure the forces they encounter during operation, reducing the risk of failure and enhancing overall safety.
Applications:
- Bridges and Skyscrapers: High yield strength alloy steel is used in beams, columns, and reinforcements to support heavy loads.
- Aircraft Components: Parts like landing gear and engine components require high yield strength to withstand operational stresses.
Table: Comparison of Yield Strength in Different Alloy Steels
Alloy Steel Grade | Yield Strength (MPa) | Key Applications | Alloying Elements |
---|---|---|---|
4140 | 655 | Gears, shafts, structural parts | Chromium, Molybdenum |
4340 | 760 | Aircraft parts, high-stress machinery components | Nickel, Chromium, Molybdenum |
8620 | 620 | Gears, crankshafts, fasteners | Nickel, Chromium, Molybdenum |
300M | 1725 | Aerospace landing gear, heavy-duty applications | Silicon, Manganese, Vanadium, Nickel |
Optimizing Alloy Steel Yield Strength for Specific Applications
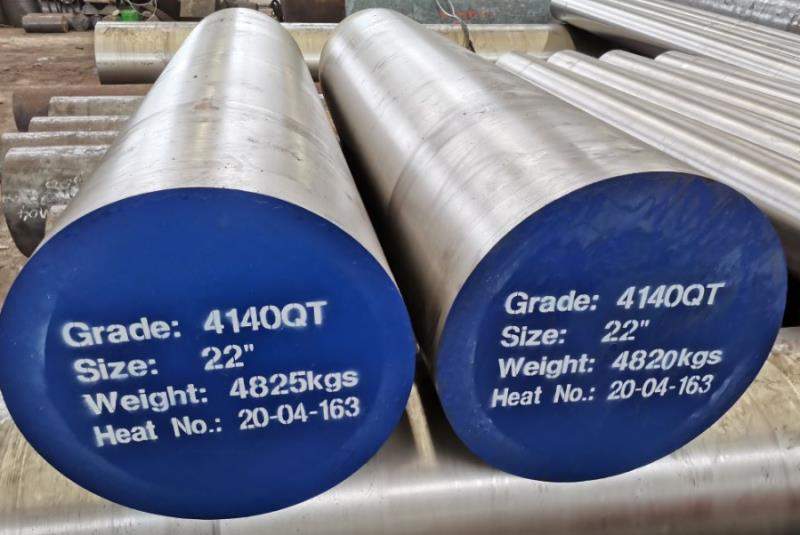
Heat Treatment Processes
Heat treatment is one of the most effective ways to optimize the yield strength of alloy steel for specific applications. Quenching and tempering are common processes used to increase yield strength by altering the microstructure of the steel. Quenching involves heating the steel to a high temperature and then rapidly cooling it, while tempering reduces brittleness by reheating the steel to a lower temperature.
Common Heat Treatments:
- Quenching: Enhances hardness and yield strength by creating a martensitic structure.
- Tempering: Adjusts hardness and toughness, balancing yield strength with ductility.
Alloy Selection for Specific Stress Conditions
Selecting the right alloy for the specific stress conditions of a project is crucial. Different alloying elements can be added to steel to achieve desired properties. For instance, adding chromium and molybdenum increases hardness and yield strength, making the steel suitable for high-stress applications.
Alloy Selection:
- High-Temperature Applications: Nickel and chromium alloys provide high yield strength and resistance to thermal stress.
- Corrosive Environments: Stainless steels with high chromium content offer good yield strength and corrosion resistance.
Conclusion
Alloy steel yield strength is a critical factor in engineering, influencing the performance, safety, and longevity of structures and components. Understanding the factors that affect yield strength and how to optimize it for specific applications is essential for engineers and designers. Whether you’re working on a construction project, manufacturing mechanical parts, or developing aerospace components, choosing the right alloy steel with the appropriate yield strength can ensure the success and safety of your project.
FAQ
What is Alloy Steel Yield Strength and why is it important?
Alloy steel yield strength is the stress level at which alloy steel begins to deform permanently. It is important because it determines the material’s ability to withstand loads without undergoing permanent deformation, which is critical in engineering applications.
How does Alloy Steel Yield Strength affect the safety of a structure?
High alloy steel yield strength ensures that structures can support heavy loads and resist deformation, thereby enhancing the safety and stability of the structure under various stress conditions.
What factors influence Alloy Steel Yield Strength?
Factors such as chemical composition, heat treatment, and manufacturing processes influence alloy steel yield strength. Alloying elements like chromium, molybdenum, and nickel play a significant role in increasing yield strength.
Why is Alloy Steel Yield Strength crucial in aerospace engineering?
In aerospace engineering, components are subjected to high stresses and extreme conditions. Alloy steel yield strength is crucial because it ensures that these components can withstand operational stresses without failing.
How does heat treatment enhance Alloy Steel Yield Strength?
Heat treatment processes like quenching and tempering alter the microstructure of alloy steel, increasing its yield strength by making it harder and more resistant to deformation.
What is the difference between Alloy Steel Yield Strength and Tensile Strength?
Alloy steel yield strength is the stress at which a material begins to deform plastically, while tensile strength is the maximum stress the material can withstand before breaking. Yield strength is critical for designing components to avoid deformation under load.
Can Alloy Steel Yield Strength be increased through alloying?
Yes, alloying elements like carbon, manganese, chromium, and molybdenum can be added to steel to increase its yield strength by enhancing its hardness and resistance to deformation.
What are the typical applications of high Alloy Steel Yield Strength?
High alloy steel yield strength is used in applications like bridges, skyscrapers, gears, shafts, and aerospace components, where materials must withstand high stress without deforming.
How is Alloy Steel Yield Strength measured?
Alloy steel yield strength is measured in units of pressure, such as megapascals (MPa) or pounds per square inch (psi), using stress-strain testing methods that determine the point at which permanent deformation occurs.
What role does Alloy Steel Yield Strength play in construction?
In construction, alloy steel yield strength ensures that materials can support structural loads, resist deformation, and contribute to the overall stability and longevity of buildings and infrastructure.